What are the steps in ventral stress fiber formation?
Recent data suggests that ventral stress fibers are created by reorganizing pre-existing dorsal stress fibers and transverse arcs [1].Other contrasting models for the formation of ventral stress fibers not covered in this resource include annealing or fusion of short actin bundles that are associated with focal adhesions [2]. Thus, the exact mechanisms for ventral stress fiber formation remain to be elucidated.
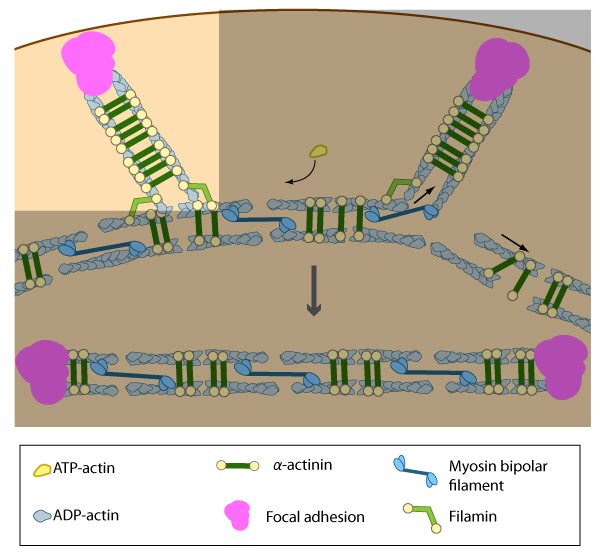
Actin filaments in ventral stress fibers are originally created either de novo or by extending pre-existing filaments. The filaments are anchored to focal adhesions and crosslinked into bundles by components of the actin linking module (e.g., α-actinin, filamin)
Association of ventral stress fibers
Similar to other cortical actin networks that are composed of actin bundles (e.g., microvilli, filopodia, stereocilia), the elongation of actin filaments in the lamellipodium would presumably contribute to the retrograde flow of actin away from the leading edge and towards the cell body, thereby fostering interactions between the dorsal stress fibers and the transverse arcs [1][3][4].
Transverse arcs filaments are thought to supply the dorsal stress fibers with filaments of mixed polarity as they are assembled; how this is achieved is relatively unknown, but based on experiments using purified components [5], permeabilized cells [6] and live cells [7][8], it has been suggested that myosin bundles may recruit the filaments and facilitate polarity sorting [9] (reviewed in [10][11]). In line with this concept, it seems reasonable to suggest that the myosin filaments may impact the dynamic interaction between the transverse arcs and dorsal stress fibers and foster their association into ventral stress fibers.
Many actin binding proteins that are found along stress fibers or at their distal adhesions (e.g., α-actinin, filamin, zyxin, talin, vinculin, espin [12], caldesmon [12]) are mechanosensitive and they are known to regulate the structure of the actin cytoskeleton (reviewed in [13][14][15][16][17][18]). Unfortunately, their exact function in stress fibers is relatively unknown. Ventral stress fibers association likely involves a dynamic interplay between number of actin binding proteins and myosin motors within the filament bundles.
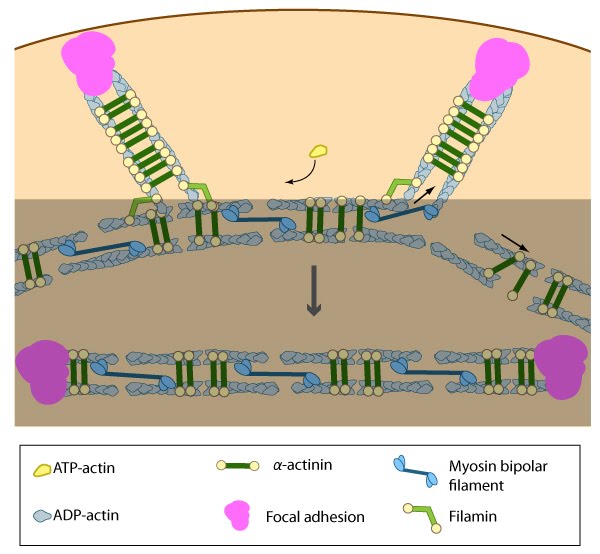
Pre-existing dorsal stress fibers interact with transverse arcs to demarcate a region of the arc that will align between the two dorsal stress fibers. Crosslinking of the actin filaments by actin binding proteins assistss this interaction. Actin subunits are exchanged throughout the stress fibers and arcs either as a result of polymerization onto the ends of actin filaments, binding to stress fiber myosin, actin treadmilling or interaction with actin-binding proteins in stress fibers (figure adapted from [16651381])
Once the bundled actin filaments in dorsal stress fibers fully interact with the transverse arc filaments, the bundles become aligned and completely ‘fuse’ to create a cohesive contractile structure [1]. Although it hasn’t been demonstrated experimentally, it is reasonable to suggest that the actin-myosin interaction and initiation of myosin II-dependent contractions will help foster ventral stress fiber alignment in a manner similar to the reorientation of lamellipodial and filopodial actin filaments during cell migration [8][7][19][20][21]. Factors that impact tension-dependent cell adhesion and actin bundle formation are also expected to be key contributors to ventral stress fiber alignment and maintenance. It should be noted that although transverse arcs formation and stability is strictly dependent upon myosin II activity, the formation and stability of ventral stress fiber appears to be somewhat less dependent [1]. Furthermore, ventral stress fibers are fairly stable and their components exhibit the slowest turnover rates relative to dorsal stress fibers and transverse arcs [1].
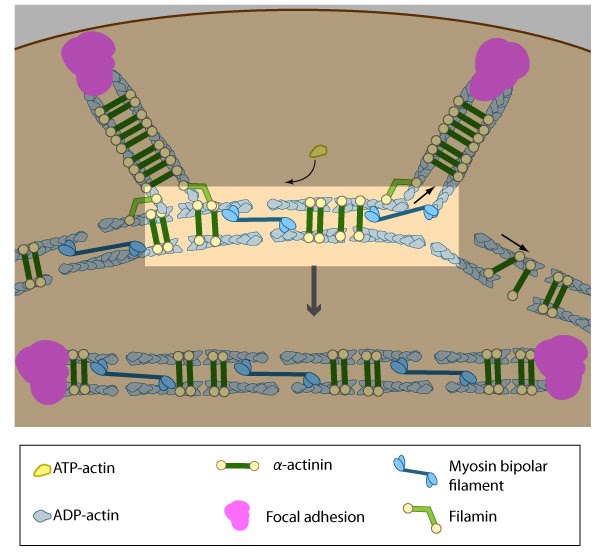
The pre-existing dorsal stress fibers interface with the transverse arcs which leaves the arc filaments aligned between the two dorsal stress fibers. Myosin bundles donated by the transverse arc continue to contract, which helps bring the fused structure into complete alignment
Contraction of ventral stress fibers
Once the actin bundles are aligned and completely ‘fused’, the completed ventral stress fiber is anchored by focal adhesions at both ends and the contractile bundles are dispersed throughout. Although stress fibers appear to contract continuously, the contractions are not uniform along their entire length [22] and the contraction strength varies with the adhesion strength (at least in muscle fibroblasts [23]). The extent of contraction also directly correlates with altered transcription of certain genes [24].
Contractile activity on a whole-cell level will influence the structural organization of the cytoskeleton and stress fiber stability/activity in ways that impact the cell morphology. For example, both contraction strength [24][25][26] and regulation of myosin II activity [27] correlate with stress fiber formation and they regulate the overall stability and integrity of stress fibers [28][29][19][30]. There is also a reciprocal relationship between the strength of contractions and changes in cell morphology: cell morphology contributes to the formation of stress fibers and the resulting magnitude of contractile force that can be generated [31], while in reverse, the extent of contractions will influence the cell morphology [24]. These examples underscore the importance of elucidating the mechanisms and molecules responsible for integrating and regulating contractile activity in stress fibers.
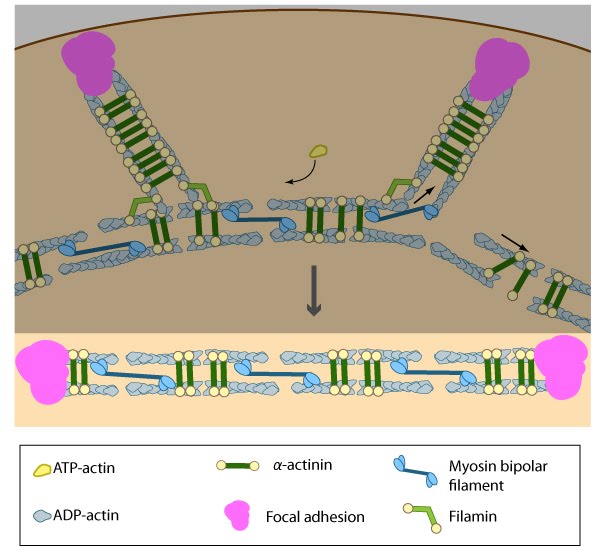
Once the actin filaments and myosin bundles are aligned, contractile activity along the filaments further organizes the bundles and brings the stress fiber to a completely ‘fused’ state: the completed ventral stress fiber is anchored by focal adhesions at both ends and the contractile bundles are dispersed throughout.
References
- Hotulainen P, and Lappalainen P. Stress fibers are generated by two distinct actin assembly mechanisms in motile cells. J. Cell Biol. 2006; 173(3):383-94. [PMID: 16651381]
- Zimerman B, Volberg T, and Geiger B. Early molecular events in the assembly of the focal adhesion-stress fiber complex during fibroblast spreading. Cell Motil. Cytoskeleton 2004; 58(3):143-59. [PMID: 15146534]
- Zhang X, Schaefer AW, Burnette DT, Schoonderwoert VT, and Forscher P. Rho-dependent contractile responses in the neuronal growth cone are independent of classical peripheral retrograde actin flow. Neuron 2003; 40(5):931-44. [PMID: 14659092]
- Lin HW, Schneider ME, and Kachar B. When size matters: the dynamic regulation of stereocilia lengths. Curr. Opin. Cell Biol. 2005; 17(1):55-61. [PMID: 15661519]
- Takiguchi K. Heavy meromyosin induces sliding movements between antiparallel actin filaments. J. Biochem. 1991; 109(4):520-7. [PMID: 1869506]
- Sanger JM, and Sanger JW. Banding and polarity of actin filaments in interphase and cleaving cells. J. Cell Biol. 1980; 86(2):568-75. [PMID: 6995468]
- Verkhovsky AB, Svitkina TM, and Borisy GG. Myosin II filament assemblies in the active lamella of fibroblasts: their morphogenesis and role in the formation of actin filament bundles. J. Cell Biol. 1995; 131(4):989-1002. [PMID: 7490299]
- Verkhovsky AB, and Borisy GG. Non-sarcomeric mode of myosin II organization in the fibroblast lamellum. J. Cell Biol. 1993; 123(3):637-52. [PMID: 8227130]
- Rhee D, Sanger JM, and Sanger JW. The premyofibril: evidence for its role in myofibrillogenesis. Cell Motil. Cytoskeleton 1994; 28(1):1-24. [PMID: 8044846]
- Small JV, Rottner K, Kaverina I, and Anderson KI. Assembling an actin cytoskeleton for cell attachment and movement. Biochim. Biophys. Acta 1998; 1404(3):271-81. [PMID: 9739149]
- Naumanen P, Lappalainen P, and Hotulainen P. Mechanisms of actin stress fibre assembly. J Microsc 2008; 231(3):446-54. [PMID: 18755000]
- Chen B, Li A, Wang D, Wang M, Zheng L, and Bartles JR. Espin contains an additional actin-binding site in its N terminus and is a major actin-bundling protein of the Sertoli cell-spermatid ectoplasmic specialization junctional plaque. Mol. Biol. Cell 1999; 10(12):4327-39. [PMID: 10588661]
- Geiger B, Spatz JP, and Bershadsky AD. Environmental sensing through focal adhesions. Nat. Rev. Mol. Cell Biol. 2009; 10(1):21-33. [PMID: 19197329]
- Carlier MF, and Pantaloni D. Control of actin dynamics in cell motility. J. Mol. Biol. 1997; 269(4):459-67. [PMID: 9217250]
- Revenu C, Athman R, Robine S, and Louvard D. The co-workers of actin filaments: from cell structures to signals. Nat. Rev. Mol. Cell Biol. 2004; 5(8):635-46. [PMID: 15366707]
- Paavilainen VO, Bertling E, Falck S, and Lappalainen P. Regulation of cytoskeletal dynamics by actin-monomer-binding proteins. Trends Cell Biol. 2004; 14(7):386-94. [PMID: 15246432]
- Insall RH, and Machesky LM. Actin dynamics at the leading edge: from simple machinery to complex networks. Dev. Cell 2009; 17(3):310-22. [PMID: 19758556]
- Sanger JW, Mittal B, and Sanger JM. Interaction of fluorescently-labeled contractile proteins with the cytoskeleton in cell models. J. Cell Biol. 1984; 99(3):918-28. [PMID: 6540785]
- Kolega J. The role of myosin II motor activity in distributing myosin asymmetrically and coupling protrusive activity to cell translocation. Mol. Biol. Cell 2006; 17(10):4435-45. [PMID: 16855019]
- Nemethova M, Auinger S, and Small JV. Building the actin cytoskeleton: filopodia contribute to the construction of contractile bundles in the lamella. J. Cell Biol. 2008; 180(6):1233-44. [PMID: 18362182]
- Anderson TW, Vaughan AN, and Cramer LP. Retrograde flow and myosin II activity within the leading cell edge deliver F-actin to the lamella to seed the formation of graded polarity actomyosin II filament bundles in migrating fibroblasts. Mol. Biol. Cell 2008; 19(11):5006-18. [PMID: 18799629]
- Peterson LJ, Rajfur Z, Maddox AS, Freel CD, Chen Y, Edlund M, Otey C, and Burridge K. Simultaneous stretching and contraction of stress fibers in vivo. Mol. Biol. Cell 2004; 15(7):3497-508. [PMID: 15133124]
- Goffin JM, Pittet P, Csucs G, Lussi JW, Meister J, and Hinz B. Focal adhesion size controls tension-dependent recruitment of alpha-smooth muscle actin to stress fibers. J. Cell Biol. 2006; 172(2):259-68. [PMID: 16401722]
- Sarasa-Renedo A, Tunç-Civelek V, and Chiquet M. Role of RhoA/ROCK-dependent actin contractility in the induction of tenascin-C by cyclic tensile strain. Exp. Cell Res. 2006; 312(8):1361-70. [PMID: 16448650]
- Saez A, Ghibaudo M, Buguin A, Silberzan P, and Ladoux B. Rigidity-driven growth and migration of epithelial cells on microstructured anisotropic substrates. Proc. Natl. Acad. Sci. U.S.A. 2007; 104(20):8281-6. [PMID: 17488828]
- Maier S, Lutz R, Gelman L, Sarasa-Renedo A, Schenk S, Grashoff C, and Chiquet M. Tenascin-C induction by cyclic strain requires integrin-linked kinase. Biochim. Biophys. Acta 2008; 1783(6):1150-62. [PMID: 18269918]
- Bao J, Jana SS, and Adelstein RS. Vertebrate nonmuscle myosin II isoforms rescue small interfering RNA-induced defects in COS-7 cell cytokinesis. J. Biol. Chem. 2005; 280(20):19594-9. [PMID: 15774463]
- Uehata M, Ishizaki T, Satoh H, Ono T, Kawahara T, Morishita T, Tamakawa H, Yamagami K, Inui J, Maekawa M, and Narumiya S. Calcium sensitization of smooth muscle mediated by a Rho-associated protein kinase in hypertension. Nature 1997; 389(6654):990-4. [PMID: 9353125]
- Rottner K, Hall A, and Small JV. Interplay between Rac and Rho in the control of substrate contact dynamics. Curr. Biol. 1999; 9(12):640-8. [PMID: 10375527]
- Goeckeler ZM, Bridgman PC, and Wysolmerski RB. Nonmuscle myosin II is responsible for maintaining endothelial cell basal tone and stress fiber integrity. Am. J. Physiol., Cell Physiol. 2008; 295(4):C994-1006. [PMID: 18701651]
- Tan JL, Tien J, Pirone DM, Gray DS, Bhadriraju K, and Chen CS. Cells lying on a bed of microneedles: an approach to isolate mechanical force. Proc. Natl. Acad. Sci. U.S.A. 2003; 100(4):1484-9. [PMID: 12552122]