What are the steps in the formation of transverse arcs?
In general, initiation of stress fiber formation is modulated by signaling cascades involving RhoA small GTPase [1] (reviewed in [2]). Most G-actin polymerization is driven by the actin polymerizing machinery at the barbed end of actin filaments, presumably at the interface between the cell membrane and the underlying filament network abutting the membrane [3][4]; these barbed ends are created by uncapping or severing existing filaments in the lamellipodium or by de novo nucleation.
The actin filaments in transverse arcs are primarily nucleated in the lamellipodia by the Arp2/3 complex at a position that is parallel to the leading edge [5]. Although effectors of RhoA signaling such as formin (e.g., mDia1) have also been suggested to nucleate actin filaments in stress fibers [6][7][8], they are not a primary contributor to transverse arc initiation and assembly [5]. It has been suggested that stress fibers may also form as a result of F-actin stabilization brought about by filament bundling and merging [9]. Consistent with this notion, actin filament bundles in filopodia were found to serve as precursors of arc-like filaments, stress fibers and graded polarity bundles [10][11].
Most of the initiated filaments in the lamellipodia have their barbed ends facing the nearest cell edge. However, the polarity of the actin filaments becomes mixed as the arcs mature towards the cell center [12]; precisely how this is achieved is unknown.
Assembly
Once initiated in the lamellipodium, the actin filaments are extended rapidly from their barbed ends. These are commonly situated at the leading edge [3], and fewer free filament ends are found in the deeper regions of the lamellipodium [12]. There is subsequent rearward transport of the actin filaments towards the cell body [12][5] (reviewed in [13]). An arc usually develops from and is positioned just beneath the dorsal surface of the lamella [12][14][15][16]. In the cases where there are periodic contractions of the lamellipodium, early arcs form over myosin filaments [17]. During their maturation however, the bundles may attain positions that are equally distributed between the ventral and middle sections of the cell [18]. Although the filaments are swept centripetally and they lack direct contact with the substrate [14], the site of initiation remains stationary relative to the substrate and the orientation of the filaments may change [16]; thus, lateral flow may foster the assembly of anti-parallel filament arrays (reviewed in [19][20]).
As the transverse arc filaments are pulled inward, they are rapidly bound and stabilized by actin binding proteins, such as α-actinin, which crosslinks the arc filaments into small bundles [5]. Similar to other cortical actin networks that are composed of actin bundles (e.g., microvilli, stereocilia), the contraction of transverse arcs at the bundle tip would presumably contribute to: the retrograde flow of actin within the bundle [21][22]; recruitment of additional proteins (e.g., filamin); and condensation of the transverse arc into larger structures as they are moved towards the cell body [5][14]. Arc-like bundles may also form from microspikes and filopodia bundles that have been recruited from the lamellipodium in the course of rearward flow [13][20].
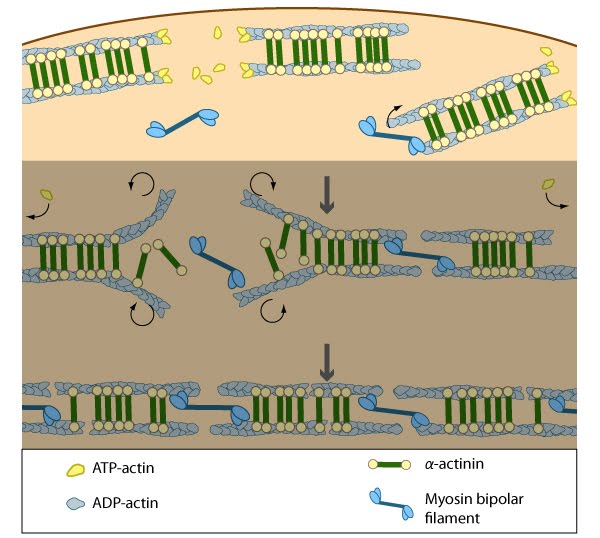
Nascent unipolar filaments are extended and are rapidly bound by actin binding proteins (e.g., α-actinin). The population of filaments steadily exchanges actin subunits and moves towards the cell body due to retrograde actin flow and actin treadmilling; the orientation of the filaments may also change with the direction of movement (figure adapted from [16651381])
Transverse arcs appear to coalesce from short actin bundles that are generated at the lamellipodial leading edge [5]. The assembly of myosin II into thick filaments also preferentially begins in the region immediately behind the leading edge as it retracts (even in the absence of net protrusion) [23][24]. The transverse arcs mature as the filaments are moved centripetally into the more stable regions of the lamellum [14][25]. As they are transported, the myosin bundles associate with the loose bundles of actin filaments and become flanked by dense bodies of α-actinin [5][26][27][28]; the spacing between the bands of α-actinin varies between cell types [29] and the organization and localization of α-actinin along the filaments is thought to follow myosin assembly [24].
Transverse arcs fully evolve from end-to-end annealing of α-actinin and myosin containing bundles [5]; end-to-end annealing has also been observed in the formation of bundles that resemble ventral stress fibers [10]. It has been suggested that tension generated by the actin-myosin arrangement and myosin II motor activity causes alignment of the actin filaments into these bundles [24][30] in a manner similar to the reorientation of lamellipodial and filopodial actin filaments during cell migration [31][10][11]. Furthermore, in order for stress fibers to be contractile, the unipolar assembly of actin filaments must change to mixed polarity bundles in mature stress fibers [32][18][12]; how this is specifically achieved is unknown, but based on experiments using purified components [33], permeabilized cells [34] and live cells [24][30], it has been suggested that myosin bundles may recruit the filaments and facilitate polarity sorting [35] (reviewed in [13][36]).
Interestingly, the assembly of transverse arcs and dorsal stress fibers appears to be connected: transverse arcs encounter dorsal stress fibers as they are transported towards the cell body via actin-myosin contractions [5].
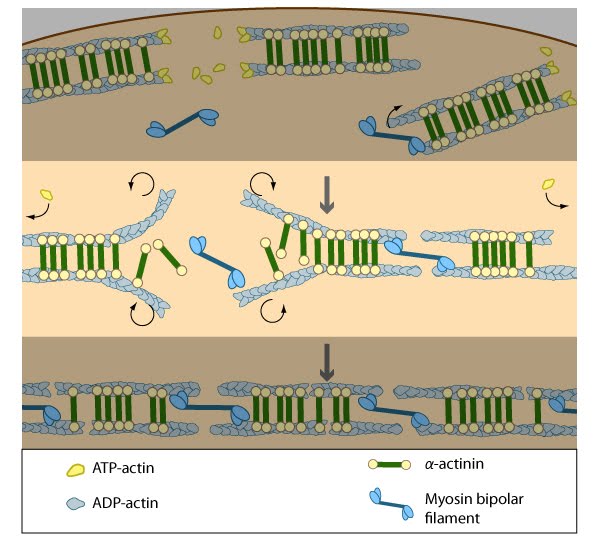
As actin filaments are transported away from the cell edge, they further evolve through end to end annealing and associate with myosin-containing bundles. The filaments undergo continued adjustment and polarity sorting to establish contractile arcs. Actin subunits are exchanged throughout the arcs either as a result of polymerization onto the ends of actin filaments, binding to stress fiber myosin, actin treadmilling or interaction with actin-binding proteins in stress fibers (figure adapted from [16651381])
As the crosslinked filaments are joined together to form long bundles, transverse arcs are fortified with myosin II and the width between alternating bands of α-actinin and myosin thick filaments is equalized [5]. Although not directly demonstrated, it is reasonable to suggest that the actin-myosin interaction and initiation of myosin II-dependent contractions fosters this alignment [37]. In line with the concept that contractile activity influences the structural organization of the cytoskeleton, the transverse arcs disassemble when the actin filaments are no longer held in place by contractile tension and the myosin II bundles [5]. Furthermore, both contraction strength [38][39][40] and regulation of myosin II activity [41] correlate with stress fiber formation and they regulate the overall stability and integrity of stress fibers [42][43][31][44]. The extent of contraction also directly correlates with altered transcription of certain genes [38]. Finally, there is a reciprocal relationship between the strength of contractions and changes in cell morphology: cell morphology contributes to the formation of stress fibers and the resulting magnitude of contractile force that can be generated [45], while in reverse, the extent of contractions will influence the cell morphology [38]. Although transverse arcs are not connected directly to focal adhesions [14], the contractile forces produced in transverse arcs can presumably be transmitted to the cell-substrate interface indirectly through the dorsal stress fibers and their associated focal adhesions. Whether this predicted force transmission actually leads to extracellular remodeling, is relatively unexplored.
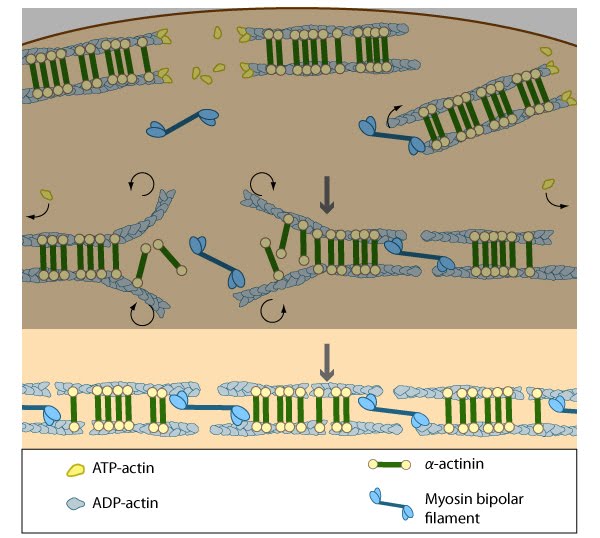
Contraction of the actin-myosin system allows the filaments to adopt a more regular structure that is contractile along its entire length; the extent of actin filament crosslinking may regulate these contractions. Myosin II motor protein activity is necessary for complete transverse arc formation (figure adapted from [16651381]).
- Ridley AJ, and Hall A. The small GTP-binding protein rho regulates the assembly of focal adhesions and actin stress fibers in response to growth factors. Cell 1992; 70(3):389-99. [PMID: 1643657]
- Ridley AJ, Schwartz MA, Burridge K, Firtel RA, Ginsberg MH, Borisy G, Parsons JT, and Horwitz AR. Cell migration: integrating signals from front to back. Science 2003; 302(5651):1704-9. [PMID: 14657486]
- Lai FPL, Szczodrak M, Block J, Faix J, Breitsprecher D, Mannherz HG, Stradal TEB, Dunn GA, Small JV, and Rottner K. Arp2/3 complex interactions and actin network turnover in lamellipodia. EMBO J. 2008; 27(7):982-92. [PMID: 18309290]
- Iwasa JH, and Mullins RD. Spatial and temporal relationships between actin-filament nucleation, capping, and disassembly. Curr. Biol. 2007; 17(5):395-406. [PMID: 17331727]
- Hotulainen P, and Lappalainen P. Stress fibers are generated by two distinct actin assembly mechanisms in motile cells. J. Cell Biol. 2006; 173(3):383-94. [PMID: 16651381]
- Watanabe N, Kato T, Fujita A, Ishizaki T, and Narumiya S. Cooperation between mDia1 and ROCK in Rho-induced actin reorganization. Nat. Cell Biol. 1999; 1(3):136-43. [PMID: 10559899]
- Tominaga T, Sahai E, Chardin P, McCormick F, Courtneidge SA, and Alberts AS. Diaphanous-related formins bridge Rho GTPase and Src tyrosine kinase signaling. Mol. Cell 2000; 5(1):13-25. [PMID: 10678165]
- Roovers K, and Assoian RK. Effects of rho kinase and actin stress fibers on sustained extracellular signal-regulated kinase activity and activation of G(1) phase cyclin-dependent kinases. Mol. Cell. Biol. 2003; 23(12):4283-94. [PMID: 12773570]
- Machesky LM, and Hall A. Role of actin polymerization and adhesion to extracellular matrix in Rac- and Rho-induced cytoskeletal reorganization. J. Cell Biol. 1997; 138(4):913-26. [PMID: 9265656]
- Nemethova M, Auinger S, and Small JV. Building the actin cytoskeleton: filopodia contribute to the construction of contractile bundles in the lamella. J. Cell Biol. 2008; 180(6):1233-44. [PMID: 18362182]
- Anderson TW, Vaughan AN, and Cramer LP. Retrograde flow and myosin II activity within the leading cell edge deliver F-actin to the lamella to seed the formation of graded polarity actomyosin II filament bundles in migrating fibroblasts. Mol. Biol. Cell 2008; 19(11):5006-18. [PMID: 18799629]
- Svitkina TM, Verkhovsky AB, McQuade KM, and Borisy GG. Analysis of the actin-myosin II system in fish epidermal keratocytes: mechanism of cell body translocation. J. Cell Biol. 1997; 139(2):397-415. [PMID: 9334344]
- Small JV, Rottner K, Kaverina I, and Anderson KI. Assembling an actin cytoskeleton for cell attachment and movement. Biochim. Biophys. Acta 1998; 1404(3):271-81. [PMID: 9739149]
- Heath JP. Behaviour and structure of the leading lamella in moving fibroblasts. I. Occurrence and centripetal movement of arc-shaped microfilament bundles beneath the dorsal cell surface. J. Cell. Sci. 1983; 60:331-54. [PMID: 6348051]
- Soranno T, and Bell E. Cytostructural dynamics of spreading and translocating cells. J. Cell Biol. 1982; 95(1):127-36. [PMID: 6890553]
- Wang YL. Reorganization of actin filament bundles in living fibroblasts. J. Cell Biol. 1984; 99(4 Pt 1):1478-85. [PMID: 6541223]
- Giannone G, Dubin-Thaler BJ, Rossier O, Cai Y, Chaga O, Jiang G, Beaver W, Döbereiner H, Freund Y, Borisy G, and Sheetz MP. Lamellipodial actin mechanically links myosin activity with adhesion-site formation. Cell 2007; 128(3):561-75. [PMID: 17289574]
- Cramer LP, Siebert M, and Mitchison TJ. Identification of novel graded polarity actin filament bundles in locomoting heart fibroblasts: implications for the generation of motile force. J. Cell Biol. 1997; 136(6):1287-305. [PMID: 9087444]
- Heath JP, and Holifield BF. On the mechanisms of cortical actin flow and its role in cytoskeletal organisation of fibroblasts. Symp. Soc. Exp. Biol. 1993; 47:35-56. [PMID: 8165576]
- Small JV, and Resch GP. The comings and goings of actin: coupling protrusion and retraction in cell motility. Curr. Opin. Cell Biol. 2005; 17(5):517-23. [PMID: 16099152]
- Zhang X, Schaefer AW, Burnette DT, Schoonderwoert VT, and Forscher P. Rho-dependent contractile responses in the neuronal growth cone are independent of classical peripheral retrograde actin flow. Neuron 2003; 40(5):931-44. [PMID: 14659092]
- Lin HW, Schneider ME, and Kachar B. When size matters: the dynamic regulation of stereocilia lengths. Curr. Opin. Cell Biol. 2005; 17(1):55-61. [PMID: 15661519]
- Kolega J, and Taylor DL. Gradients in the concentration and assembly of myosin II in living fibroblasts during locomotion and fiber transport. Mol. Biol. Cell 1993; 4(8):819-36. [PMID: 8241568]
- Verkhovsky AB, Svitkina TM, and Borisy GG. Myosin II filament assemblies in the active lamella of fibroblasts: their morphogenesis and role in the formation of actin filament bundles. J. Cell Biol. 1995; 131(4):989-1002. [PMID: 7490299]
- McKenna NM, Meigs JB, and Wang YL. Exchangeability of alpha-actinin in living cardiac fibroblasts and muscle cells. J. Cell Biol. 1985; 101(6):2223-32. [PMID: 4066755]
- Langanger G, Moeremans M, Daneels G, Sobieszek A, De Brabander M, and De Mey J. The molecular organization of myosin in stress fibers of cultured cells. J. Cell Biol. 1986; 102(1):200-9. [PMID: 3510218]
- Lazarides E, and Burridge K. Alpha-actinin: immunofluorescent localization of a muscle structural protein in nonmuscle cells. Cell 1975; 6(3):289-98. [PMID: 802682]
- Sanger JW, Sanger JM, and Jockusch BM. Differences in the stress fibers between fibroblasts and epithelial cells. J. Cell Biol. 1983; 96(4):961-9. [PMID: 6339529]
- Sanger JW, Mittal B, and Sanger JM. Interaction of fluorescently-labeled contractile proteins with the cytoskeleton in cell models. J. Cell Biol. 1984; 99(3):918-28. [PMID: 6540785]
- Verkhovsky AB, and Borisy GG. Non-sarcomeric mode of myosin II organization in the fibroblast lamellum. J. Cell Biol. 1993; 123(3):637-52. [PMID: 8227130]
- Kolega J. The role of myosin II motor activity in distributing myosin asymmetrically and coupling protrusive activity to cell translocation. Mol. Biol. Cell 2006; 17(10):4435-45. [PMID: 16855019]
- Begg DA, Rodewald R, and Rebhun LI. The visualization of actin filament polarity in thin sections. Evidence for the uniform polarity of membrane-associated filaments. J. Cell Biol. 1978; 79(3):846-52. [PMID: 569662]
- Takiguchi K. Heavy meromyosin induces sliding movements between antiparallel actin filaments. J. Biochem. 1991; 109(4):520-7. [PMID: 1869506]
- Sanger JM, and Sanger JW. Banding and polarity of actin filaments in interphase and cleaving cells. J. Cell Biol. 1980; 86(2):568-75. [PMID: 6995468]
- Rhee D, Sanger JM, and Sanger JW. The premyofibril: evidence for its role in myofibrillogenesis. Cell Motil. Cytoskeleton 1994; 28(1):1-24. [PMID: 8044846]
- Naumanen P, Lappalainen P, and Hotulainen P. Mechanisms of actin stress fibre assembly. J Microsc 2008; 231(3):446-54. [PMID: 18755000]
- Chrzanowska-Wodnicka M, and Burridge K. Rho-stimulated contractility drives the formation of stress fibers and focal adhesions. J. Cell Biol. 1996; 133(6):1403-15. [PMID: 8682874]
- Sarasa-Renedo A, Tunç-Civelek V, and Chiquet M. Role of RhoA/ROCK-dependent actin contractility in the induction of tenascin-C by cyclic tensile strain. Exp. Cell Res. 2006; 312(8):1361-70. [PMID: 16448650]
- Saez A, Ghibaudo M, Buguin A, Silberzan P, and Ladoux B. Rigidity-driven growth and migration of epithelial cells on microstructured anisotropic substrates. Proc. Natl. Acad. Sci. U.S.A. 2007; 104(20):8281-6. [PMID: 17488828]
- Maier S, Lutz R, Gelman L, Sarasa-Renedo A, Schenk S, Grashoff C, and Chiquet M. Tenascin-C induction by cyclic strain requires integrin-linked kinase. Biochim. Biophys. Acta 2008; 1783(6):1150-62. [PMID: 18269918]
- Bao J, Jana SS, and Adelstein RS. Vertebrate nonmuscle myosin II isoforms rescue small interfering RNA-induced defects in COS-7 cell cytokinesis. J. Biol. Chem. 2005; 280(20):19594-9. [PMID: 15774463]
- Uehata M, Ishizaki T, Satoh H, Ono T, Kawahara T, Morishita T, Tamakawa H, Yamagami K, Inui J, Maekawa M, and Narumiya S. Calcium sensitization of smooth muscle mediated by a Rho-associated protein kinase in hypertension. Nature 1997; 389(6654):990-4. [PMID: 9353125]
- Rottner K, Hall A, and Small JV. Interplay between Rac and Rho in the control of substrate contact dynamics. Curr. Biol. 1999; 9(12):640-8. [PMID: 10375527]
- Goeckeler ZM, Bridgman PC, and Wysolmerski RB. Nonmuscle myosin II is responsible for maintaining endothelial cell basal tone and stress fiber integrity. Am. J. Physiol., Cell Physiol. 2008; 295(4):C994-1006. [PMID: 18701651]
- Tan JL, Tien J, Pirone DM, Gray DS, Bhadriraju K, and Chen CS. Cells lying on a bed of microneedles: an approach to isolate mechanical force. Proc. Natl. Acad. Sci. U.S.A. 2003; 100(4):1484-9. [PMID: 12552122]